NeuroNex News
November 2023, Issue #4
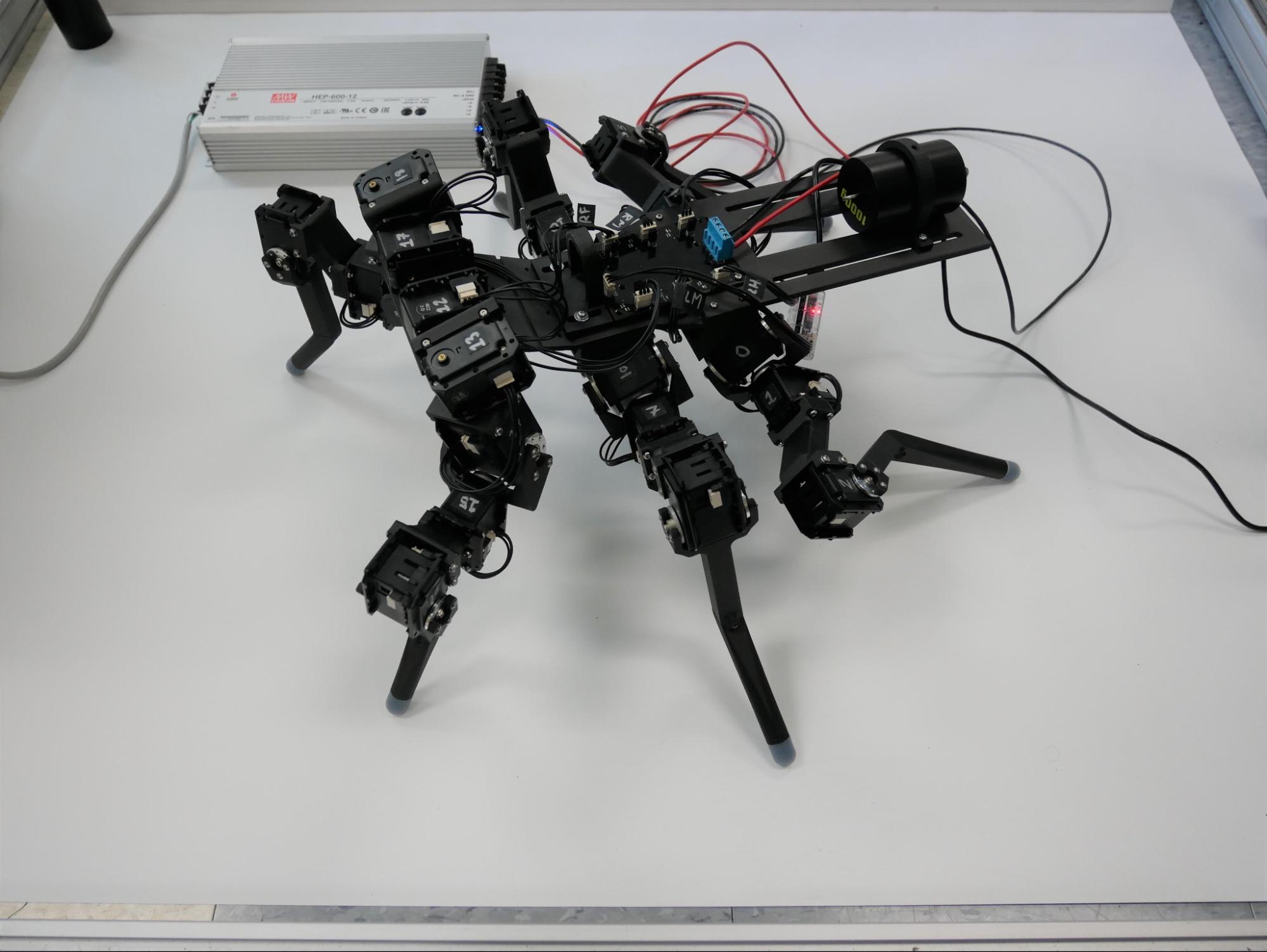
This dynamically scaled robot, Drosophibot II, is modeled after a walking fruit fly, Drosophila melanogaster. Developed by Clarus Goldsmith and Nicholas Szczecinski at West Virginia University, Drosophibot II was built using the phase shift framework that considers which forces are dominant during movement, thus determining their role in neural control.
If ever a horse and a stick insect were in a race together (and what a short race that would be!), they would probably look the same on the outside—leg muscles engaged, contracted to propel the body forward, then extended for the next stride. What we wouldn’t be seeing, however, are four forces at play in the two differently sized participants—gravity, elasticity, viscosity, and inertia. These forces matter when it comes to controlling movement, but the size and speed of the animal help determine not only which of these forces is dominant but also how the force behaves.
In large animals, like the horse, gravity—the force that makes things fall, and inertia—the resistance to change in motion—play a big part given the animal’s large mass and speed. On the other hand, a smaller animal like a stick insect is less concerned with those two forces; its movement is dominated by elasticity—the ability to stretch and bounce back, and viscosity—internal friction in its joints.
When it comes to identifying which force is dominant in any given animal, researchers have traditionally relied on various ratios of two of the four forces; however, those numbers fall short because no one number can account for the role—whether minimal or dominant—of all four forces depending on the size or speed of the animal.
As a solution, NeuroNex researchers, part of the Communication, Coordination, and Control in Neuromechanical Systems (C3NS) project, have developed a method to use all four forces to quantify which force is dominant in the movement of animals across varied sizes and speeds.
Read all about their research on NeuroNex News.
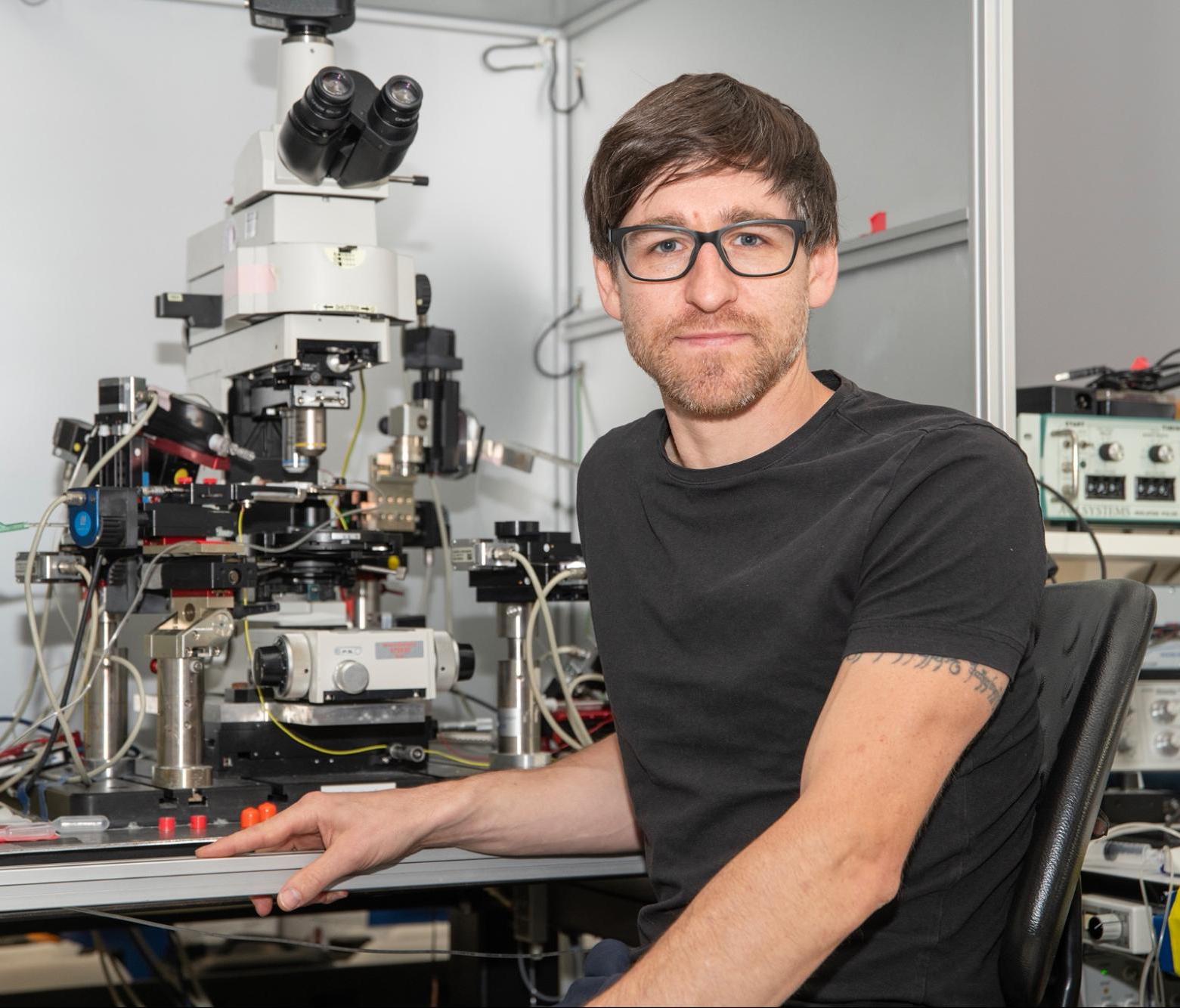
Tobias Ackels in his newly established lab at the University of Bonn. Image credit: Rolf Mueller/University Hospital Bonn
In August 2023, Tobias Ackels, a former trainee with the NeuroNex Project, Odor2Action: Discovering Principles of Olfactory-Guided Natural Behavior, moved from the UK to Germany to set up his own lab at the University of Bonn—one of Germany’s biggest neuroscience hubs. There, he's part of a dynamic community of scientists dedicated to pioneering research and seeking collaborative innovation. Against this backdrop, however, lies the challenge of setting up a new lab. Aside from the bureaucracy and red tape that are part and parcel of any setup, there’s the prospect of interviewing potential research candidates—an exciting endeavor for Ackels; and the need to purchase equipment. In fact, one of Ackels’ early investments was a two-photon microscope, which costs hundreds and thousands of dollars; a purchase he referred to as terrifying because of the enormous cost. And then, of course, there are countless other logistical and administrative tasks—such as applying for an animal research license to study mice. For Ackels, a busy researcher and father of two young children, all of this translates to long—albeit fulfilling—days. Early Years of Neuroscience Originally from the small town of Bitburg in western Germany, Ackels did his PhD in neuroscience at Aachen University, where he focused on signaling mechanisms in the mouse olfactory system. (Ackels also met his wife—a fellow neuroscientist—at Aachen University.) He then moved to London for a postdoc with Andreas Schaefer, Co-PI on the NeuroNex Odor2Action project and professor at the Francis Crick Institute. There, Ackels explored how mammals perceive and process natural sensory stimuli and collaborated with other members of the NeuroNex network. The turning point for Ackels’ career occurred in 2021, when his research on how mice process and respond to the temporal structure of fast odor dynamics. was published in Nature. That success as first author led him to apply for a European Research Council Starting Grant—tailored for early-career scientists looking to work independently. At that point, Ackels was in search of a long-term setup with stability and job security, and so the idea to establish his own lab was born. “Having the option to really be able to stay in one place to raise your kids and, at the same time, be in a scientifically stimulating environment was very important for me,” says Ackels. “So that became an integral part of the entire decision process.” Almost two years later, Ackels, his wife—who works at University College London’s Sainsbury Wellcome Centre—along with their three-month-old and two-year-old were headed for Bonn. New Beginnings Three months into setting up the lab, Ackels has just started to build his team and has already found a talented graduate student. Their research will focus on how mammals process temporally dynamic smells that they pick up from the environment and how that information elicits behavior. The team will specifically study how mice rely on their heightened sense of smell, particularly since they’re crepuscular—creatures that mostly move around in darkness or semi-darkness. The researchers will explore how mice can use their olfactory sense to gain spatial information about their sensory environment. In a natural setting this would, for example, translate to determining how close or far away a particular scent might be. “Mice can detect whether an odor comes from the same source or from different sources,” says Ackels.” In somewhat simplistic terms, this means that an animal is able to tell that a food source is over here and a predator is over there.” What researchers don’t know, however, is how the olfactory system actually makes use of these dynamic odor signals—how those scents are being processed in the brain and the actual underlying mechanisms. Once that’s clear, the next step would be to use that information to understand why the mouse responds in a certain way. “In order to get to the bottom of this, you have to use state-of-the-art techniques,” says Ackels. “You want to record the activity of neurons in different parts of the olfactory system with high precision. You also want to monitor the navigation strategy of the animal at high temporal and spatial resolution—exactly how the animal moves; how it turns its head; where it puts its paws.” All of this will be studied in a custom-built arena under controlled lab conditions. For that, Ackels not only needs to get his animal research license application approved (this tends to take several months) but also continue to build his team of researchers. In the meantime, Ackels continues to stay in touch with the NeuroNex Odor2Action team. “The NeuroNex groups are working on questions that are highly related to what I have been working on and will continue to work on over the next years,” he says. “Therefore, it’s great for me to maintain contact with those colleagues because the exchange and interactions are really priceless.”
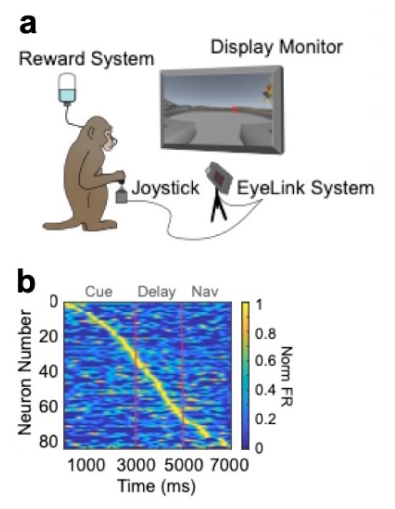
Here, we see how certain neurons in the macaque’s brain are activated in a specific order as the animal plans and executes movements. As the macaque works through the computer-generated maze (a), the graph (b) shows how neurons—represented by the red lines—are activated at different times during the trial, representing first imaginary trajectories and then physical navigation through the maze.
The Fabric of the Primate Neocortex and the Origin of Mental Representations is studying advanced thinking processes in marmosets and macaques—specifically how neurons across the brain create and retain representations of information in working memory (our “mental sketch pad”) without any sensory stimulation from the environment. This is an international NeuroNex project, with researchers based in the US, Canada, and Germany. Much of the team’s research is focused on brain cells in the prefrontal cortex, a complex region at the front of the brain. Researchers are learning that these cells have specialized connections, physiological properties, and contain more molecules that help support working memory and abstract cognition in the primate brain. For instance, the primate cortex contains areas organized into two distinct streams that respectively process and hold spatial information—where an object is located, for example, and non-spatial information—features such as the color or shape of an object. Prefrontal cortical neurons also have extensive local connections that allow them to excite each other to retain information in working memory stores. The neurons themselves have special properties, and the Neuronex team has been building a database of ex vivo recordings, carried out in the lab, that help capture these unique physiological properties of prefrontal neurons in macaques and marmosets, for direct comparison with the Allen Institute for Brain Science’s database of the mouse and human cortex. There are also differences in the molecular composition of the neurons, with macaques and humans showing steep gradients across the cortical hierarchy from the first stages of sensory processing in the primary sensory cortex up to the highest stages in the prefrontal cortex. For example, neurons in the prefrontal cortex contain more calcium-related proteins that are needed to support their sustained activation during working memory. These findings suggest that primate brains have evolved in their size; their molecular and cellular complexity; as well as how their cells connect, all contributing to the capacity for abstract thought.