Researchers Consider Four Forces to Study Animal Movement
If ever a horse and a stick insect were in a race together (and what a short race that would be!), they would probably look the same on the outside—leg muscles engaged, contracted to propel the body forward, then extended for the next stride. What we wouldn’t be seeing, however, are four forces at play in the two differently sized participants—gravity, elasticity, viscosity, and inertia. These forces matter when it comes to controlling movement, but the size and speed of the animal help determine not only which of these forces is dominant but also how the force behaves.
In large animals, like the horse, gravity—the force that makes things fall, and inertia—the resistance to change in motion—play a big part given the animal’s large mass and speed. On the other hand, a smaller animal like a stick insect is less concerned with those two forces; its movement is dominated by elasticity—the ability to stretch and bounce back, and viscosity—internal friction in its joints.
When it comes to identifying which force is dominant in any given animal, researchers have traditionally relied on various ratios of two of the four forces; however, those numbers fall short because no one number can account for the role—whether minimal or dominant—of all four forces depending on the size or speed of the animal.
As a solution, NeuroNex researchers, part of the Communication, Coordination, and Control in Neuromechanical Systems (C3NS) project, have developed a method to use all four forces to quantify which force is dominant in the movement of animals across varied sizes and speeds.
Their work was recently published in PNAS Nexus.
As part of this research, the authors introduce the “phase shift,” which measures the difference in timing between the force exerted by muscles—the actuator force—and the actual movement of the limb. The resulting number, say researchers, can show which force is dominant in an animal’s movement.
That means, for example, that if a person were to stretch their arm such that it was horizontal to the ground and their muscles were fully engaged, the phase shift calculation would point to a high dominance of elastic forces. However, if the outstretched arm now dropped to the side and muscles were still at maximum capacity, inertia would be the dominant force.
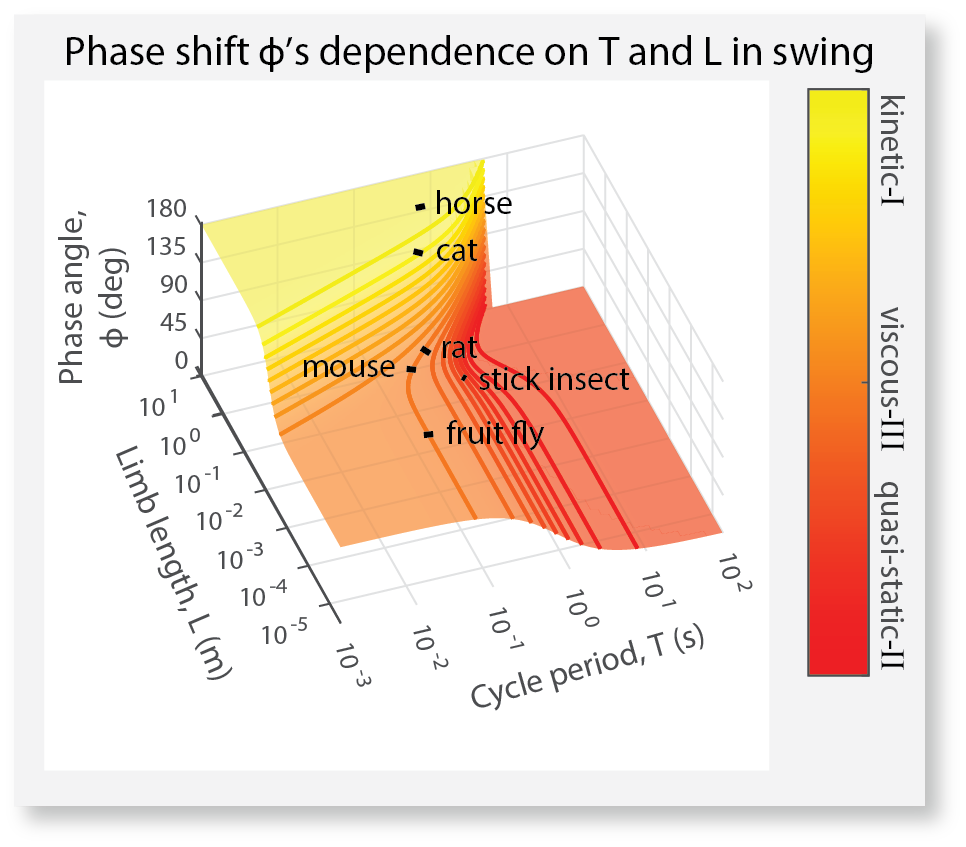
“This timing of the behavior relative to when the forces are maximum, gives us one thing that we can measure,” says Gregory Sutton, professor at the University of Lincoln’s School of Life Sciences in the UK and lead co-author on the recently published paper. “If you know what the muscle forces are and know what the joint angles are, you can just figure out what the timing is between them, and that will tell you whether there’s inertial, viscous, or elastic.”
But these calculations don’t just help with identifying the dominant force. They can also predict how a limb will respond to unexpected changes or perturbations. So, if a horse steps into a hole, knowing the phase shift can help predict the impact and effect on its limb.
“Once you know which force is important, from a biology standpoint, you know the mechanical world the nervous system is navigating to control the motion,” says Sutton. If you’re in the inertial realm, you have to worry about getting knocked over; you have to worry about slowing down your joints to avoid getting hurt. A horse at full gallop that hits a hole can shatter its leg. And the nervous system must control the legs such that this never happens or, if it starts to happen, the nervous system has to respond immediately.
The phase shift can also help understand when a particular muscle will be activated in response to a particular activity by measuring a muscle’s electrical activity—also known as electromyography or EMG. Researchers compared EMG recordings of a horse and stick insect and found that the phase shift could predict the timing of muscle activation in both creatures.
From an engineering point of view, this information can help researchers build robots that are dynamically similar. With the right ratio of inertia and elastic springs, for instance, researchers can build a cat-sized robot that mimics the movements and mechanics of a fruit fly—something that Nicholas Szczecinski, assistant professor at West Virginia University’s Mechanical and Aerospace Engineering Department and lead co-author on the paper, is currently doing with fellow researcher Clarus Goldsmith and others on his team. As part of the effort, researchers are using this information to change the speed of the fruit fly robot while experimenting with different levels of elasticity and viscosity in its motors.
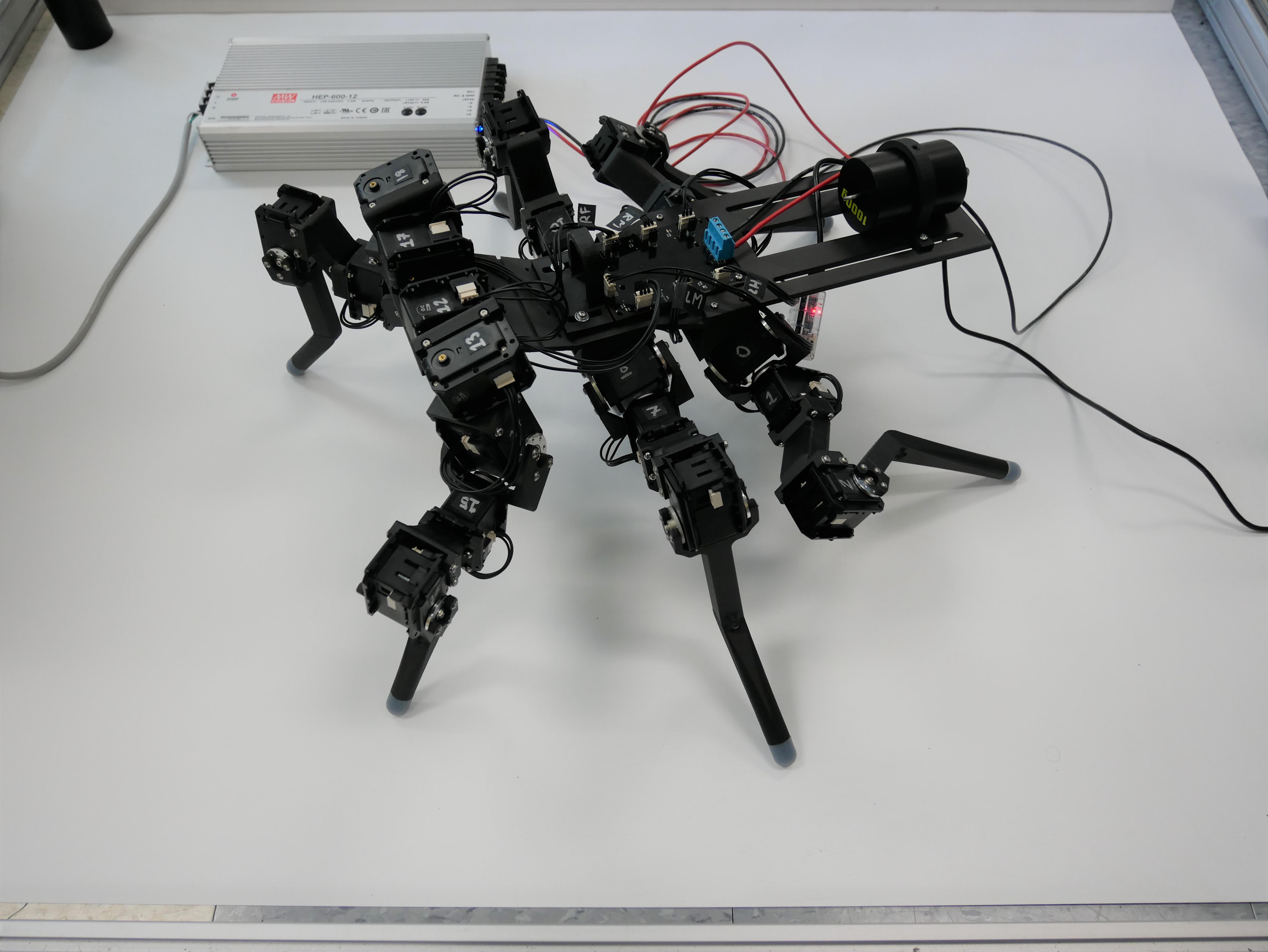
This team of engineers and biologists exemplifies the power of the NeuroNex Network’s approach to research. “By creating collaborations between engineers and biologists from around the world, the Network makes it possible to put together ideas that make both neuroscience and biorobotics stronger,” says Roger Quinn, engineering professor at Case Western Reserve University and author on the paper.
In fact, these recent findings have potential medical applications as well. When it comes to neural prosthesis or powered prosthetics, we know our hands have an elastic dominated kind of setup, which allows it to move with a certain flexibility that’s lacking in our hips for instance; instead, the hip oscillates like a pendulum. “There’s no one size fits all dynamic model of any given joint,” says Szczecinski. “If you were replacing one person’s hand and another person’s knee or hip, you might take different things into consideration and make it more natural to control.”
According to Hillel Chiel, professor at Case Western Reserve University’s College of Arts and Sciences and last author on the paper, “Finding general principles in biology is hard, so it is exciting to develop a framework that can unify a vast range of animal behaviors, and potentially lead to more flexible, adaptive robots.”