NeuroNex Community Presents Research at 4th NeuroNex Investigator Meeting
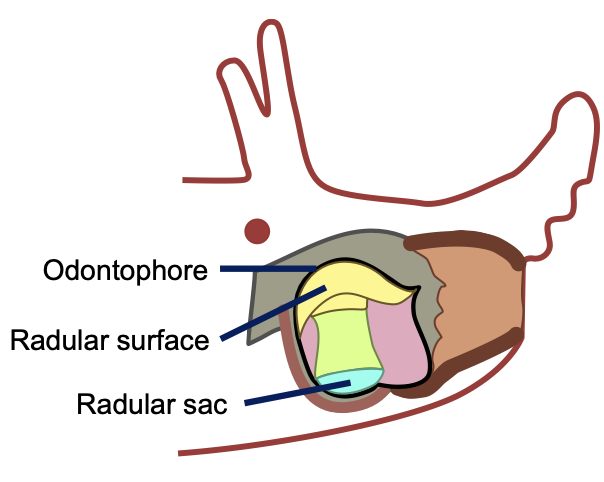
Eating is a complex behavior that, if truly understood, helps shed light on how the nervous system functions. Researchers at Carnegie Mellon University have been studying the feeding mechanisms of the sea slug, Aplysia californica as part of collaborations with researchers at Case Western Reserve University within the Communication, Coordination, and Control in Neuromechanical Systems NeuroNex Network. By modeling how slugs feed in simulation and with bioinspired robots, the team seeks to better understand how neural control and biomechanics work together in the slugs ability to grasp and eat food. But to build good models, the team needs to know how materials in the slug deform when muscles pull on them. Recently, the research team launched a pilot study to test and analyze the role of non-muscular tissue, specifically within the odontophore–a food grasping structure within the slug’s mouth. As part of this effort, they developed material models that will help understand how variations in odontophore shape can affect overall feeding behavior. “In the C3NS Network, we are really interested in how the brain and the body interact to produce useful behavior,” says Vickie Webster-Wood, Assistant Professor at Carnegie Mellon University. “Of course, the materials that the body is made of play an intricate role in this, and so it is important that our models capture these mechanics."
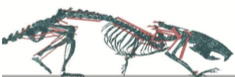
In order to improve a balance control model that mimics human data, researchers at Portland State University have developed a two-stage process to select neural model parameters. First, they used a functional subnetwork approach to attain a starting point and bring the model in the neighborhood of a solution. Next, researchers deployed a particle swarm optimization algorithm in order to quickly locate the optimal solution. This pairing of techniques helps overcome limitations inherent to each.
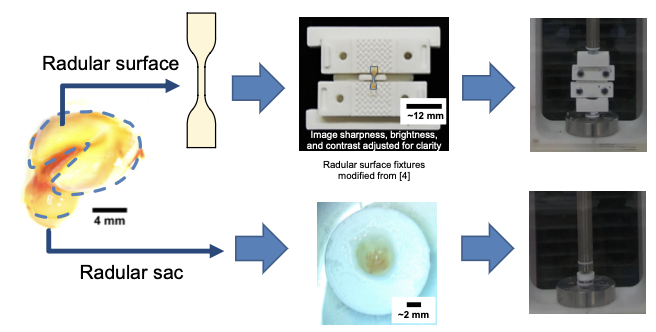
Researchers at Université Laval have developed a deep learning model to help generate super-resolved microscopy images, called Task-Assisted GAN (TA-GAN). This model is optimized to accurately translate low-resolution images (such as confocal) into their super-resolution counterpart (such as STimulated Emission Depletion or STED). Results show that TA-GAN generated STED images can be used to guide microscopy image acquisition, especially for live-cell imaging. Researchers included the TA-GAN in the acquisition loop of the confocal and STED microscopes to provide microscopists real-time information about the nanoscale content of low-resolution acquisitions–without actually having to acquire super-resolved images at each step.
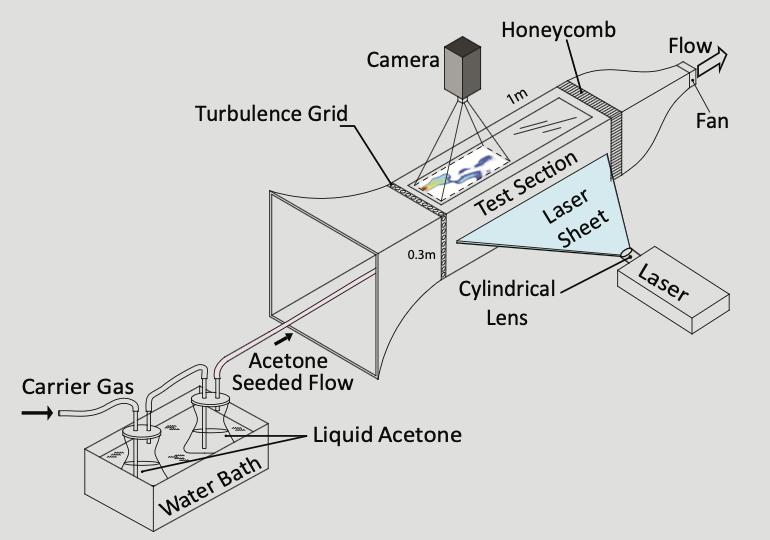
How does an animal trace the source of an odor? Typically, it relies on its built-in tools such as antennae or the nose. But tracing the source of an odor involves more than just sensation; it involves action as well such as waving its antennae or sniffing. These actions can influence how odors mix with the air, and either help—or hurt—the animal’s ability to find the odor source. To truly understand the complexity of this integrative process of sensation and action, researchers at Cornell University and the University of Colorado, Boulder have combined mathematical and computational methods with physical measurements of how odors spread. They showed that action and sensation can be combined to provide a more accurate localization of an odor source. What’s more, researchers found that different strategies are optimal for odors that spread in open space versus near a surface. “Localization of an odor source is crucial for animal behavior and for human activities, such as environmental monitoring,” says Jonathan Victor, Neurology and Neuroscience Professor at Weill Cornell Medical College. “This work shows that successful localization of an odor is best achieved by combining sensation with actions that modify the way that odors mix with the air.”
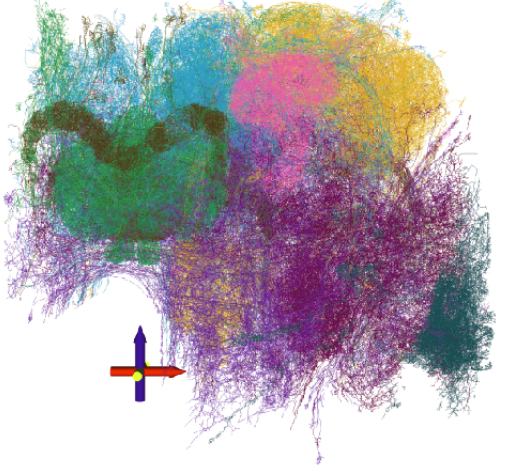
Two-photo calcium imaging helps monitor the activity of distinct neurons in the brain. However, when it comes to estimating functional connectivity with the help of that data, the critical signal–neuronal firing–is represented by sparsely occurring spikes in the activity trace. Since existing probabilistic graphical models tend to find relationships with respect to mean values and not extremes, functional connectivity estimates from these models often capture associations between measurement noise rather than spiking activity. As a solution, researchers at the Baylor College of Medicine and Rice University recently developed the Subbotin graphical model—designed to estimate functional connectivity from two-photon calcium imaging data under the probabilistic graphical modeling framework. This method captures information that lies in rare extreme outliers more effectively than existing graphical modeling techniques, using a single-step approach that eliminates the potential misclassification of spikes that can occur when applying data-preprocessing. “The Subbotin graphical model will be applied in the future on larger scale calcium imaging data sets to help study patterns and structures in functional neuronal network architectures,” says Andersen Chang, Postdoctoral Associate at the Baylor College of Medicine.
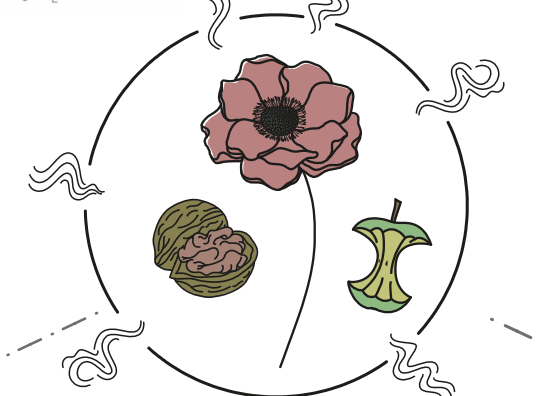
The Odor2Action Network is a NeuroNex International Network bringing together over 70 international scientists from three countries—the US, UK, and Canada. The project’s aim is to achieve an end-to-end understanding of how brains organize and process information from odors to guide adaptive behaviors. Using a team science approach, researchers focus on collaborations that will push the field of olfaction forward at an accelerated pace. The team has expertise in a variety of fields such as neurophysiology, neurobiology, molecular genetics, mathematics, computational neuroscience, behavior, ecology, fluid mechanics, and beyond.
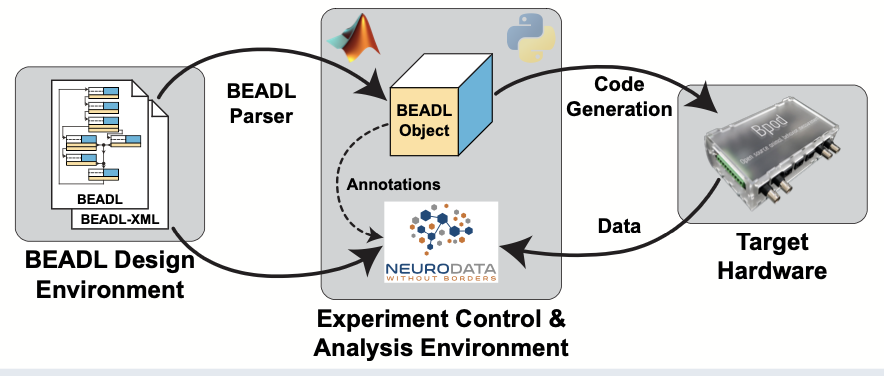
While the field of neuroscience has made great strides in developing technologies to measure and manipulate brain activity, not as much progress has been made in developing universal methods to describe a lot of those behaviors. In response, researchers at Washington University in St. Louis have developed a framework to describe and implement behavioral tasks in neuroscience experiments along with a data format to store the results after executing the behavioral task. Introducing BEADL, the BEhavioral tAsk Description Language, which defines behavioral tasks as an abstract state machine and includes easy-to-understand flow diagram layouts and associated design software. This graphical representation of a behavioral task can be exported as a corresponding XML-based definition to use as a formal description to generate code for task execution environments. An additional Neurodata Without Borders or NWB extension allows for storing of behavioral data, capturing both the BEADL task description together with the behavioral output of a subject.
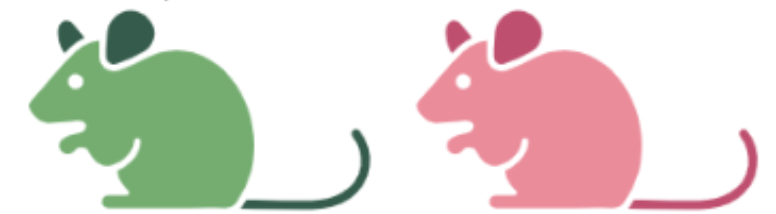
BioLuminescent OptoGenetics (BL-OG) enables genetically targeted bimodal activation of neurons. Optogenetic elements are activated chemogenetically through application of a small molecule luciferin that is oxidized by a luciferase enzyme tethered to the optogenetic element, thereby emitting bioluminescence light and opening a channelrhodopsin. The same elements are activated optogenetically by physical light sources (LED) in standard optogenetic experiments. Researchers at Central Michigan University have taken advantage of this tool by utilizing the chemogenetic access to systematically and non-invasively enhance activity levels in pan-neocortical layer 5 pyramidal neurons during early postnatal brain development, and by utilizing the optogenetic access to the same neurons in the same animals as adults to dissect the electrophysiological consequences of developmental hyperexcitation.
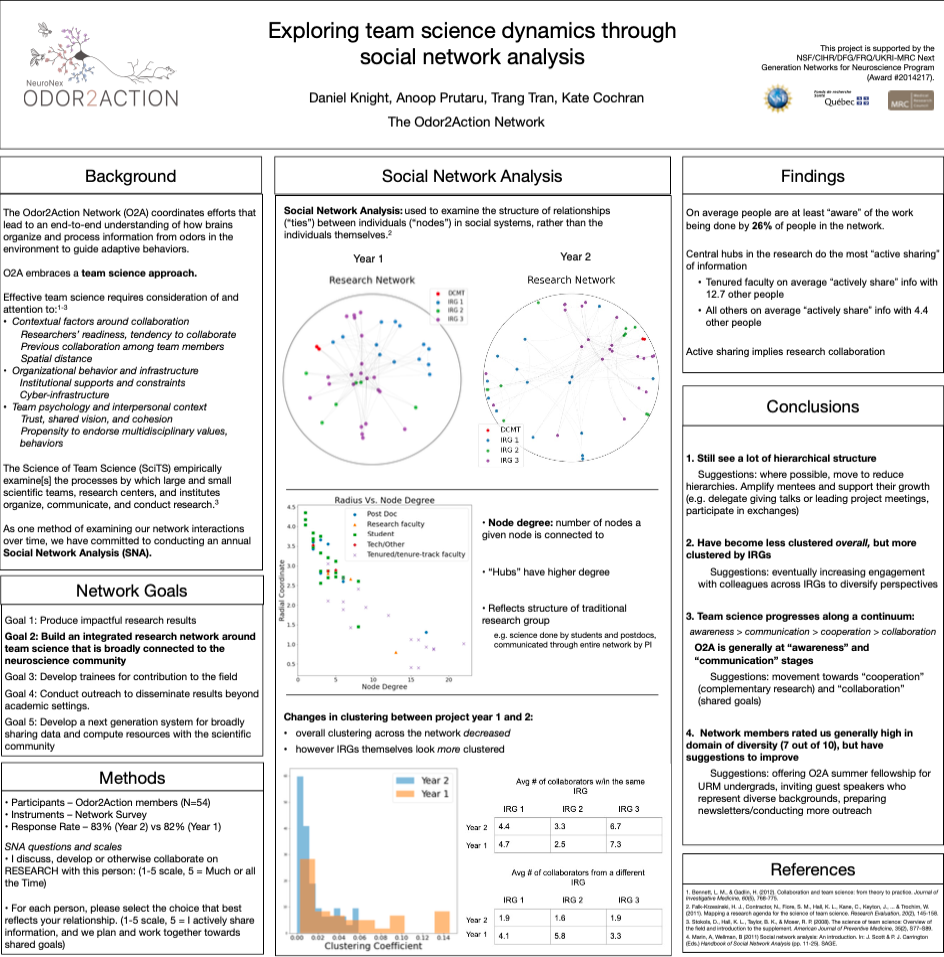
The Odor2Action Network embraces a team science approach to understanding how brains organize and process information from odors in the environment to guide adaptive behaviors. To better understand the team science dynamics within this Network over time, researchers are conducting an annual social network analysis. They anticipate that their ongoing analyses will inform changes to their approach to collaboration and will contribute to the growing body of knowledge around effective team science. “We’re finding that each year we conduct these analyses on our group dynamics, we’re able to detect subtle, measurable changes in our structure and then craft a narrative of how we’ve evolved,” says Kathryn Cochran, Project Manager for the NeuroNex Odor2Action Network. “Right now these narratives help us implement strategies that ideally position us to achieve the goals we are setting, and down the road we can look back at these data holistically to glean more about what conditions lead to successful team science.
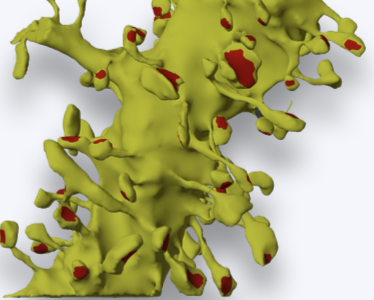
Researchers at Salk Institute, Pittsburgh Supercomputing Center, Texas Advanced Computing Center, and UT Austin’s Center for Learning and Memory have built an intuitive graphical user interface for aligning serial section electron micrographs (ssEM) using Signal Whitening Fourier Transforms (SWiFT). AlignEM-SWiFT is a graphical extension of SWiFT-IR, a proven suite of image registration programs developed by computer scientist Arthur Wetzel at the Pittsburgh Supercomputing Center.
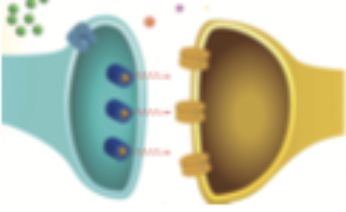
At the Bioluminescence Hub, researchers systematically develop and disseminate novel and powerful bioluminescent tools for brain science while creating educational curricula and outreach events for all ages. Bioluminescence has several advantages as an imaging tool. This approach provides high signal-to-noise ratio output without the need for fluorescent light input. Keys to making this approach more broadly useful are generating brighter output, optimizing tools for practical imaging of these signals, and effective targeting of indicator sensitivity to relevant neural fluctuation ranges. Recent Hub progress includes development of a new series of particularly bright calcium indicators, indicators integrated into calcium channels, optimization of light-weight microscopes for bioluminescent imaging, and optical synapses.
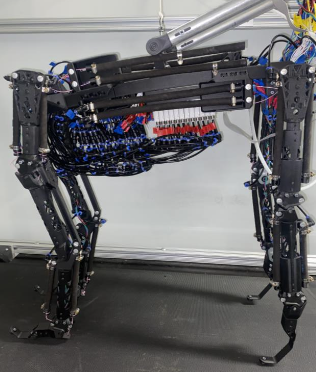
This poster depicts the collaborations that take place within the C3NS NeuroNex network. C3NS consists of four independent research groups (IRGs). IRG1 functions as the modeling group guiding three experimental IRGs (2-4). Each of the three experimental IRGs study animals within different dynamical regimes. IRG2 studies the fly, Drosophila melanogaster, whose walking behavior is dominated by viscous and elastic forces. IRG3 studies Aplysia californica, whose feeding behavior is dominated by elastic forces. IRG4 studies small mammals, whose walking behavior is dominated by inertial and elastic forces.
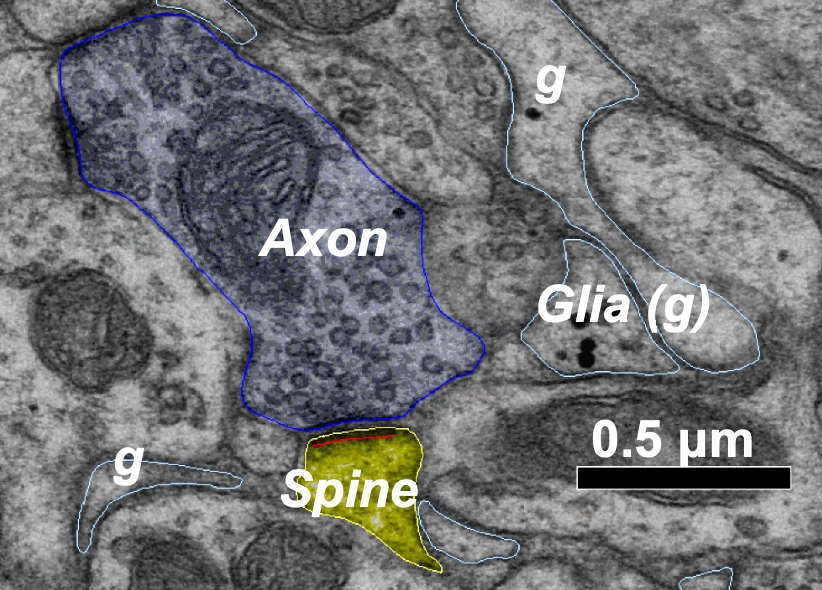
Astrocytes are important for information processing in the brain, however, how are they impacted by a change in the structure or function of a neural synapse–the junction between two nerve cells in the brain? In an effort to answer that question, NeuroNex-funded researchers are studying how long-term potentiation (LTP), the enhancement of synaptic transmission due to specific activities, and long-term depression (LTD), the reduction of synaptic transmission–both widely accepted mechanisms of learning and memory alter astrocyte processes. Specifically, they’re looking at if and how these long-term changes in neural plasticity caused by LTP and LTD impact the hippocampal dentate gyrus, a region of the brain that helps form long-term memory. "This research will help build a more complete picture of how other cell types beyond just neurons contribute to information processing in the brain,” says Andrea Nam, Graduate Research Assistant at the University of Texas at Austin. “These results may also extend to have important implications on our understanding of the mechanisms underlying different brain disease states."
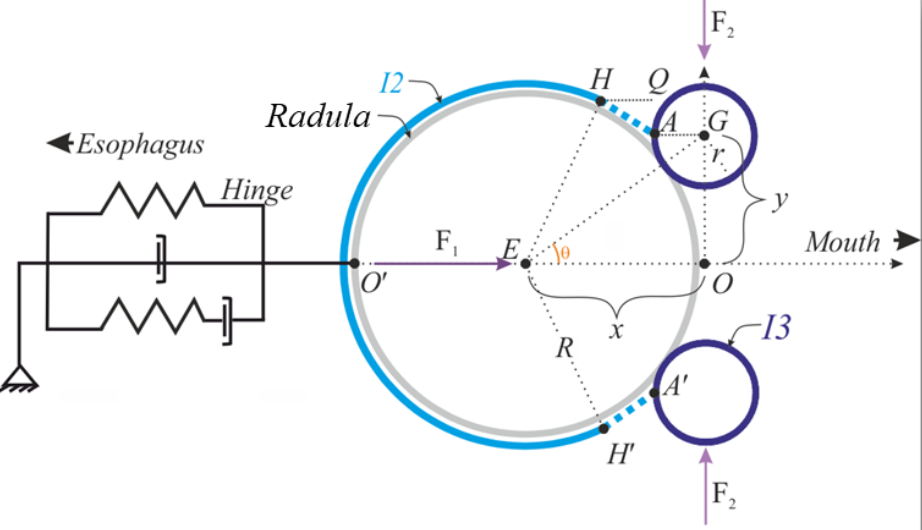
This poster presents recent progress in the Communication, Coordination, and Control NeuroNex network towards understanding how ascending and descending neural signals control slow and steady behaviors in the sea slug, Aplysia californica. To study sea slug neuromechanics, researchers in the C3NS collaborative network develop new models of the sea slug feeds, both for the animal's body and brain. These models are tested in simulation and in robotic platforms and compared to data collected from actual animals.
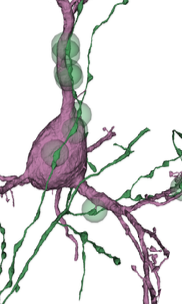
In the mammalian cortex, information processing spans a collection of diverse neurons arranged in a series of six layers, often described as a cortical column. However, we still lack a full picture of what cell types exist and how they communicate with one another. Here, NeuroNex-funded researchers use electron microscopy to reconstruct nearly 1,400 neurons across all layers of the mouse visual cortex with synaptic resolution. Including synapse-related properties such as average synapse size along with traditional morphological features helps partition excitatory neurons into novel subtypes that reflect differences in the local cortical wiring. What’s more, researchers found that inhibitory neurons are organized into "connectivity groups," small collections of distinct cell types that inhibit the same excitatory subtypes. The team’s dense survey also allowed them to identify a new class of inhibitory neuron that almost exclusively targets basket cells, which could be important for controlling the gain of cortical activity.
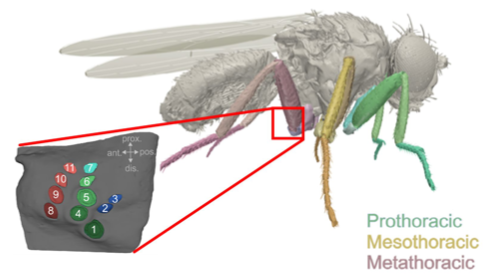
This poster presents recent progress in the Communication, Coordination, and Control NeuroNex network towards understanding how ascending and descending neural signals control rapid, low inertia walking of the fruit fly, Drosophila melanogaster. To study fruit fly neuromechanics, researchers in the C3NS collaborative network develop new models of the kinematics, dynamics, and material properties of the legs as the animal walks. Experiments in fruit flies quantify the effect of silencing or activating sensory organs; identify descending neurons that activate or deactivate specific behaviors, e.g., walking backward; and classify ascending neurons by the type of signal they encode (e.g., joint rotations, leg forces) and how they branch within the nervous system. The team uses robotic models of the fruit fly to consolidate these results and compare the model to data collected from animals.
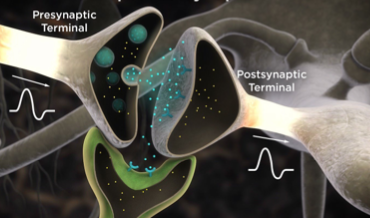
Interluminescence is a novel and non-invasive technology that relies on bioluminescence–light produced by a chemical reaction–to study communication in the brain. While interluminescence has typically been focused on neuron-to-neuron communication, researchers at Central Michigan University have expanded its focus to include neuron-non neuron communication and ultimately general cell-to-cell communication. With this wider application, interluminescence could be used to study a neuron-muscle partnership or perhaps to target the neuroendocrine system. Here, researchers have applied the Interluminescence strategy to control neuron-muscle communication at the neuro-muscular junction via this ‘optical synapse’.
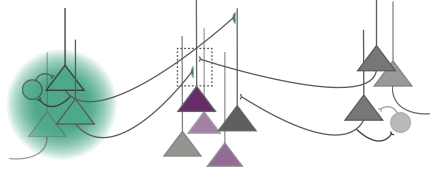
Thalamic nuclei in the brain are not only responsible for relaying incoming sensory information to the neocortex but are also involved in mediating communication between cortical areas and modulating sensory and motor signals based on behavioral contexts and brain states such as attending versus ignoring or sleeping versus waking. Oscillatory synchrony within neural populations and across cortical regions also plays a part in both communication between brain regions as well as context dependent modulation of cortical processing. A computational model from this NeuroNex-funded group proposes that one of these oscillatory rhythms is driven by bursting activity simultaneously onto the proximal and distal dendrites of cortical layer 5 pyramidal neurons. Based on known anatomical connections, the team hypothesized that bursts of spikes from the posterior medial nucleus (POm) of the thalamus are responsible for generating these rhythmic events in the cortex. "The alteration of 'typical' oscillatory brain signatures are correlated with a host of brain disorders such as Parkinson's disease, schizophrenia, and Autism Spectrum Disorders," says Jeremy Murphy, a Postdoctoral Research Associate at Brown University. "Understanding the precise brain circuits involved in the generation of these oscillations will provide greater insight into the etiologies of these disorders."
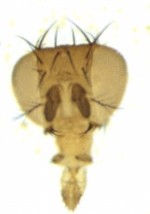
While many insect species play a critical part as pollinators or in their role as secondary food sources, others can be detrimental in their ability to spread disease or destroy agricultural crops. In an effort to better understand and perhaps modify the behavior of some insects, NeuroNex-funded researchers are developing molecular and imaging tools that will help shed light on the neural function of potentially any insect species regardless of existing genomic information.
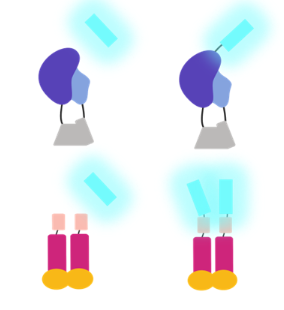
While researchers have typically used external sources of light to study the brain, many are turning to bioluminescent-optogenetic or BL-OG, a new, non-invasive way to control communication between neurons by using biological light emitted from one cell to activate light-sensing channels in another cell. But this method of studying cell communication isn’t restricted to neuroscience; instead it can be used to activate any photosensory protein, thus allowing researchers to manipulate numerous light-mediated functions in cells.
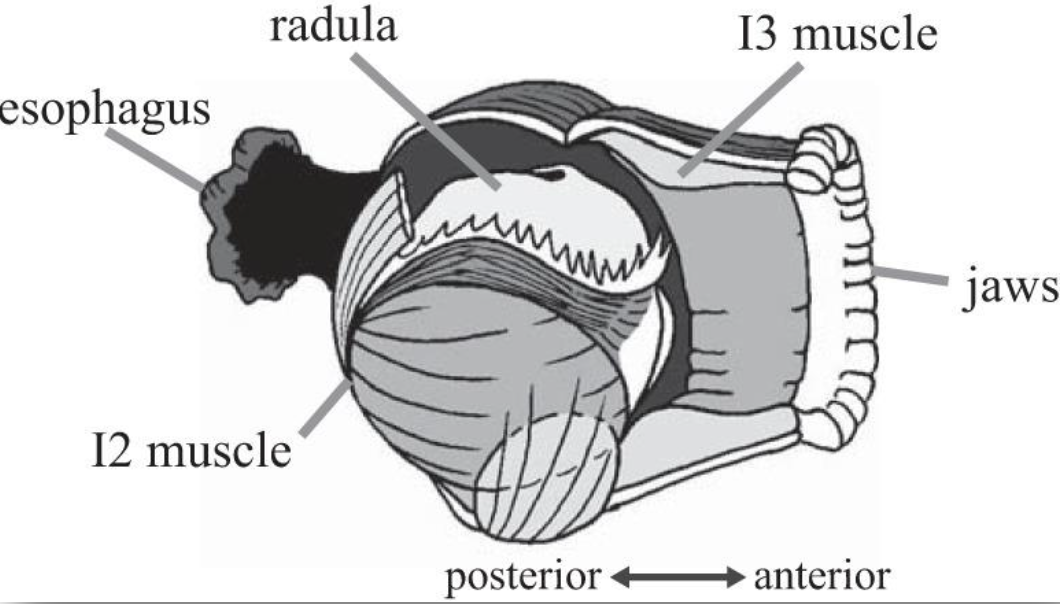
Behavior is produced through a combination of neural and muscle activity. Understanding and modeling how the nervous system controls geometrically complex muscular structures is extremely challenging. In addition, this complexity often leads to another challenge for simulations: long computational times. In response, this C3NS NeuroNex group is studying the mechanical control of the geometrically complex muscular structures controlling feeding in the marine mollusk, Aplysia californica. The feeding apparatus (buccal mass) moves under the influence of several muscle forces activated by neural commands from the buccal ganglion. The team has modeled this system with four components: 1) the odontophore, a spherical grasping structure; 2) the I2 muscle, a sheet of muscle posterior to the odontophore; 3) the I3 muscle, a large toroidal muscle anterior to the odontophore; and 4) a hinge which attaches the ventral odontophore to I3. However, because this complex system moves slowly, the team recently proposed an alternate solution based on a quasi-static relationship between motion and neural activation.
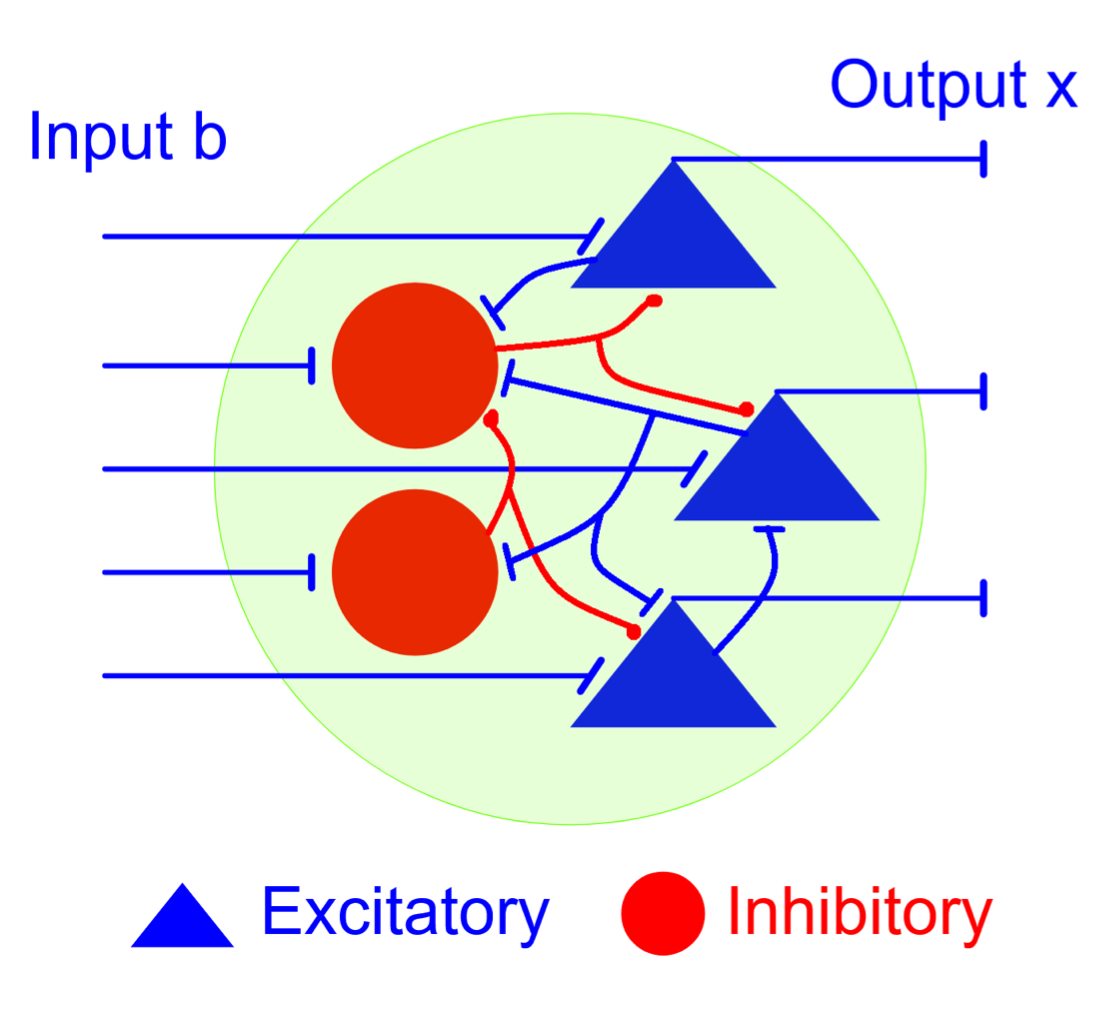
Relating connectivity features to the function of a recurrent neuronal network is a long-standing problem that still remains at large. In response, this NeuroNex-funded team describes the combinatorics of equilibria and steady states of neurons in recurrent networks that satisfy Dale’s law–the principle that a neuron performs the same chemical action at all of its synapses. Their research shows that the combinatorial code of a Dale network can be characterized in terms of two properties: the network connectivity graph and a spectral condition on the synaptic matrix. The team found that in the weak coupling regime the combinatorial code depends only on the connectivity graph, and not on the particulars of the synaptic strengths. They also proved that the combinatorial code of a weakly coupled network is a sublattice, and provide a learning rule for learning a sublattice in such a network.